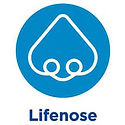
INTRODUCTION
Health-care strategies are currently oriented towards non-invasive techniques for an early diagnosis. Chemical analysis seems to be a good answer to accomplish both prevention, a fundamental requirement for an efficient treatment of the disease, and non-invasivity. Many diseases and intoxications are accompanied by characteristic odors, which can be especially perceptible to the breath. In these last three decades a series of instruments, such as gas chromatography [GC] and mass spectrometry, have been used for the identification of molecules responsible of typical odors occurring in specific diseases. Their recognition can provide diagnostic clues, guide the laboratory evaluation, and affect the choice of immediate therapy.
Breath is fundamentally composed of nitrogen, oxygen, carbon dioxide, water and inert gases. In addition, mixtures of numerous compounds occur at concentrations in the ppb–ppm range. Some of these compounds could be markers of a specific pathology[1]. The number of these compounds is higher than 200, as shown in the 70s by Pauling et al. in one of his first work about breath analysis by GC[2]. Experiments performed in the last two decades pointed out a number of volatile substances probably connected to certain diseases[3]. The correlation between VOCs and diseases seems to be due to the fact that several organs contribute to the composition of breath, which is expected to be rich in information coming from biochemical processes triggered by different pathologies[4].
GC is very accurate, but is expensive and not always, its sampling and assaying processes are complicated and time consuming, while its results require expert interpretation. A less expensive and more portable alternative is electronic nose. Electric noise is also cheaper and faster, and often used outside of medicine in fields related to food, chemistry, fragrances, security, and environment.
Recently, electronic noses have been developed as a non-invasive, painless and simple screening method for early medical diagnosis to recognize characteristic smells from diseases and bacteria cells.
Sensors employed in e-nose
Gas molecules interacting with solid-state sensors, by absorption, adsorption or chemical reactions with films thickness of the sensors. The sensor appliance finds the physical and/or chemical modifications caused by these processes, and these modifications are calculated as an electrical signal.
Conducting polymer compounds, intrinsically conductible polymers and metal oxides, are three of the most conventionally utilized classes of sensing materials in conductivity sensors. These materials work on the premise that a modification in some feature, as consequence of interacting with a gas/smell, leads to a change in sensor resistance. The sensing material is deposited over interdigitated or two aligned electrodes which create the electrical connections through which the relative resistance modification can be measured. The heater is needed when metal oxides are utilized as the sensing material, because very high temperatures are asked for capable action of metal oxide sensors. Conducting polymer compounds give more benefits than other materials when utilized as gas sensor. High discrimination in array sensors can be simply carried out using these materials because of the wide range of polymeric compounds available on the market. This is due to the fact that different compounds produce dissimilar levels of responses to a given odor. Conducting polymer compounds are also cheap enough and easy to arrange. Sensors prepared using these compounds can run in conditions of high relative humidity and also show highly linear profiles for a wide spectrum of gases[5]. No heater is needed when sensors made of these materials run at room temperature. This is an essential advantage with portable battery powered e-nose systems, because heater would significantly step up the power of the system at room temperature. The signal conditioning circuitry required for these sensors is quite simple because only a resistance change is being measured. The main disadvantage of using conducting polymer compound as e-nose sensors is their short life span which leads to sensor drift; moreover these compounds are unsuitable for detecting some gases. For example, carbon-polymer compounds are not sensitive to trimethylamine (TMA) for fish smell applications. Also intrinsically conducting polymers have many benefits when used in e-nose systems. Increased discrimination when developing sensor arrays can simply be achieved with these compounds as a wide range of conducting polymers are available on the market[6]. ICP sensors operate at room temperature[7], so simplifying the required system electronics. Conducting polymers show a good answer to a wide range of analytes and have fast feedback and recovery times mainly for polar compounds. Problems connected to intrinsically conducting polymer sensors include poorly understood signal transduction mechanisms, difficulties in resolving some types of analytes, high sensitivity to humidity and the sensor response can drift with time. The manufacturing techniques for these sensors can be not easy and time –consuming and a wide differences in the characteristics of the sensor can occur from batch to batch[8]. Finally, the lifetime of these sensors can be quite short, about 9-18 months, because of the polymer oxidation[9]. The main advantages of metal oxide sensors are the quick response and recovery times, depending on the temperature and the level of mutual action between sensor and gas[10]. Thin film metal oxide sensors are small and quite cheap, they have lower power consumption than thick film sensors and can be directly integrated into the measurement circuitry[11]. However, they have many disadvantages because of their high operating temperature which causes more power consumption over sensors made of materials other than metal oxides. As a result, no handheld e-nose systems have been made utilizing metal oxides sensors[12]. Besides, they suffer from sulphur poisoning due to irreversible binding of compounds containing sulphur to the sensor oxide[13] and ethanol can also blind the sensor from other volatile organic compound (VOC), gases.
Artificial Neural Network
The sensor signals obtained in the e-nose are saved and then the data analysis process is started. Improvements in the performance of the available data analysis techniques are an important topic of research on electronic nose development.
Artificial Neural Network (ANN) offer potential advantages in non-parametric pattern recognition, classification, and distinction among multicomponent chemical sensor systems[14]. The main characteristic of an ANN model is whether it needs direction in learning or not. Based on the way they learn, all artificial neural networks can be divided into two learning categories: supervised and unsupervised. In supervised learning, a preferred output result for each input vector is necessary when the network is trained. An ANN of the supervised learning kind, such as the multilayer perception, uses the objective result to conduct the formation of the neural parameters. Hence, by learning the behavior of the process under study it is possible to build the neural network. In unsupervised learning, the working out of the network is totally data driven and no target results for the input data vectors are provided. An ANN of the unsupervised learning type, such as the self-organizing map, can be utilized for clustering the input data and find features inherent to the problem. The ANNs are computer programs based on a simplified model of the brain; they reproduce its logical operation using a collection of neuron-like entities forming networks to perform processing. ANN programs are multipurpose and with suitable training, a single program could solve a number of problems.
There is a significant number of Pattern-Recognition algorithms used for e-nose data processing and continuous developments are being made in this regard. The basic requirement for neural networks is their ability to follow the brain’s pattern-recognition methods. The wide success of neural networks can be attributed to some key factors. Neural networks, with their significant capability to derive meaning from complicated or indefinite data, can be used to remove patterns and detect development that are too difficult to be noticed by either humans or computer techniques. A trained neural network can work as an ‘‘expert’’ in the class of information it has been given for analysis.
STATE OF THE ART
Commercial Electronic Nose Instruments
Many electronic noses are commercially available today and they have a wide range of applications in several markets and industries, ranging from food processing, industrial manufacturing, quality control, environmental protection, security, safety and military applications to several pharmaceutical medical, microbiological and diagnostic applications. A summary of some of the currently and most widely used electronic noses with manufacturers models available and technological basis are listed in Wilson, A.D.; Baietto, M.[15], but they are limited applications in breath analysis. One reason for this might be the design of commercial e-noses for broad applications rather than for breath analysis specifically. Generally the chemical sensors found in commercial e-noses are not particularly sensitive to the biomarkers and compositions in human breath.
The potential or future developments of go-ahead e-nose applications is colossal as researchers in many fields of scientific investigations and industrial development become more aware of the capabilities of the electronic nose. The common trend is toward the development of electronic noses for peculiar purposes or a fairly narrow range of applications. This strategy increases e-nose efficiency by minimizing the number of sensors needed for discriminations, decreasing instrument cost, and allowing for greater portability through miniaturization.
Scientific and technological challenges and ways and methods to overcome them
Recently e-noses have gradually been used in medicine for the diagnosis of lung cancer, diabetes and tuberculosis. The exhaled breath of patients with lung cancer has different unique characteristics that can be identified with an e-nose made with the use of polymer sensory array. The results provide available options to the concept of using the e-nose for administration and detecting lung cancer[16]. Blatt et al. obtained remarkable detection results of lung cancer diagnosis using MOS sensors[17]. Other work regarding lung cancer diagnosis by an electronic nose are also availalbe [18],[19]. In another research, Guo et al. conducted a study on diagnosis of diabetes, renal disease, and airway inflammation using MOS sensor array, with the result that breath analysis can be employed for early diagnosis of many diseases[20]. Arend et al. explored the prospect of two different e-noses to differentiate between sputum headspace samples from TB-patients and non-TB patients[21]. With the help of e-nose a sample test is done and the early diagnosis of tuberculosis followed by appropriate treatment will reduce the trouble of tuberculosis.
OBJECTIVES AND OVERVIEW
The research project aims at exploring the applications of neural networks applied to electronic nose. The general objective of work will lead to the development of a portable biomedical instrument able to diagnose, in a noninvasive manner, diseases in humans.
The hybrid (nonspecific and specific sensors) configuration of the sensors will increase the sensitivity and the stringency of the analyte’s analyses. Nonspecific chemical sensors will be made of nanostructured films of conducting polymers, phthalocyanines, carbon nanotubes and inorganic nanoparticles. Specific sensors will be constructed by molecular imprinting technique in polymer films and metal oxides. The analysis will mainly focus on identifying low levels of substances known to be biomarkers of diabetes, lung cancer and pulmonary tuberculosis, respectively.
The specific objectives are: (1) to verify the capacity of the sensors to discriminate the biomarkers, (2) to improve the discrimination due to contribution of ANN’s, (3) to implement the algorithms in an embedded hardware platform, (4) to develop the portable instrument, (5) to conduct in vitro and in vivo experiments.
RESEARCH METHODOLOGY AND APPROACH
As already mentioned, the commercial electronic nose instruments have limited applications in the breath analysis and a portable and inexpensive instrument, capable of discriminate the presence of biomarkers in low concentrations, is not yet available and could provide an early diagnosis of illness and save many lives, especially in poor areas. A classical example is Tuberculosis. This disease is largely curable, and treatment for TB is considered one of the most cost-effective health interventions. Tuberculosis claims the lives of approximately 1.3 million people per year[22]. Our goal is to develop the electronic nose widely available in poor, remote areas where illness often breeds and spreads, devastating so many lives.
ORIGINALITY AND INNOVATIVE ASPECTS OF THE RESEARCH PROGRAM
Our work will extend into three parallel directions not yet routed in reported researches:
-
Improvement of sensors technology with the development of longer-life and stable sensors. Moreover, the usage of hybrid (nonspecific and specific) sensors configuration, measured under AC, is desirable in order to obtain both selectivity and sensitivity.
-
Improvement of classification techniques in which we emphasize the importance of evaluating other classification algorithms (as support vector machines, Bayesian approaches or other topologies of ANN), as well as the use of different algorithms to select the best subgroup of features for each classifier, instead of using ranking techniques based on statistical tests. The training of the ANN will be done also in presence of noise, since it has been demonstrated that ANNs can compensate humidity, drift and temperature variation phenomenons[23] that affect olfactory signals.
-
Cheapness (by in-house developed, low cost sensors) and portability (using microcontroller or DSP rather than expensive and bulky external processing systems).
INTERDISCIPLINARY AND MULTIDISCIPLINARY ASPECTS
​
The topic of research concerns the bioelectronics, which establish a synergy between electronics and biology. The bioelectronics requires research that crosses disciplines, such as electrical engineering, biology, chemistry, physics, and materials science. The transition of the results of this multidisciplinary research to commercial products will be expedited through collaboration at early stages between the electronics and the biomedical device industry, along with the academic communities.
​
IMPACT
​
The impact of the research findings (rapid detection of illness such as diabetes, lung cancer and pulmonary tuberculosis), would be of enormous importance in clinical, both for the non-invasiveness, and for the drastic reduction of costs related to instrumental analysis. In this perspective, the realization of a low cost and commercial tool has a considerable potential.
​
PROTOTYPE
​
Lower figure shows the device prototype realized in the Biomedical Laboratory of the faculty of Physics at the Altai State University. It is constituted by seven different gas sensors, one transducer of humidity and temperature, and by a Microchip PIC microcontroller, which digitally converts the measured values of gas concentration of every transducer and computes the temperature and humidity correction. The microcontroller (Microchip PIC18F2520) is a high performance RISC CPU, based on a modified Harvard architecture operating up to 5 MIPS with analogue features. The seven gas sensors used to develop the electronic nose system (Henan Hanwei Electronics Co) are MQ-2 (General combustible), MQ-3 (Alcohol), MQ-4 (Natural gas, Methane), MQ-5 (LPG, Natural gas, Coal gas), MQ-6 (LPG, Propane), MQ-8 (Hydrogen), MQ-135 (Air Quality Control).
The Hanwey sensors require a simple heating and measuring circuit to work. The heating circuit was built inside the sensor and needs to be heated to high temperature to be sensitive to a particular gas present in the air. In the presence of a detectable gas, the sensor conductivity increases and the measuring circuit converts the change in conductivity to a voltage signal. Temperature and humidity are acquired by the Sensirion SHT-75 transducer that integrates two sensor elements and signal processing in a compact format providing a fully calibrated digital output. A unique capacitive sensor element is used for measuring relative humidity while temperature is measured by a band-gap sensor. Both sensors are seamlessly coupled to a 14bit analog to digital
converter and a serial interface circuit. The device works in slave mode and its acquired and computed data are available by means of a host/slave ASCII serial communication protocol (RS232-C, 115.2 kbps). A RS232 transceiver is used to interface an external host which operates at RS232-C levels. This is necessary in order to reduce the communication errors and to extend the host/slave distance.
​
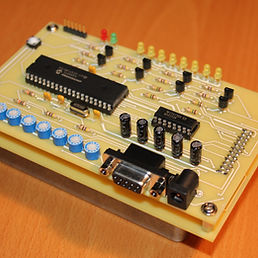
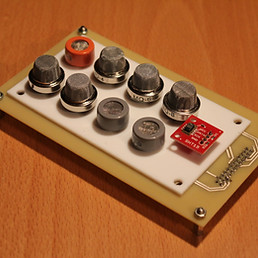
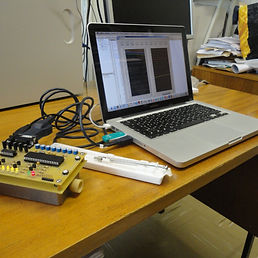
THE PROJECT
[1] M. Phillips, J. Herrera, S. Krishnan, M. Zain, J. Greenberg, R. Cataneo, Variation in volatile organic compounds in the breath of normal humans, J. Chromatogr. Biomed. Appl. 729 75–88 (1999).
[2] L. Pauling, A.B. Robinson, R. Teranishi, P. Cary, Quantitative analysis of urine vapour and breath by gas–liquid partition chromatography, Proc. Natl. Acad. Sci. U.S.A. 68 2374–2376 (1971).
[3] M. Phillips, Breath test in medicine, Sci. Am. 267 74–79 (1992).
[4] W. Miekisch, J.K. Schubert, G. Noeldge-Schomburg, Diagnostic potential of breath analysis - focus on volatile organic compounds, Clin. Chim. Acta 347 25–39 (2004).
[5] Munoz, B.C., Steinthal, G. and Sunshine S., Conductive polymer-carbon black composites-based sensor arrays for use in an electronic nose”, Sensor Review, Vol. 19 No. 4, pp. 300-305 (1999).
[6] Albert, K.J. and Lewis, N.S., Cross reactive chemical sensor arrays, Chem. Rev, Vol. 100, pp. 2595-2626 (2000).
[7] Shurmer, H.V. and Gardner, J.W., Odour discrimination with an electronic nose, Sensors and Actuators B, Vol. 8, pp. 1-11 (1992).
[8] Nagle, H.T., Gutierrez-Osuna, R. and Schiffman, S.S., The how and why of electronic hoses, IEEE Spectrum, Vol. 35 No. 9, pp. 22-31 (1998).
[9] Schaller, E., Bosset, J.O. and Escher, F., Electronic noses and their application to food”, Lebensmittel-Wissenschaft und-Technologie, Vol. 31 No. 4, pp. 305-316 (1998).
[10] Penza, M., Cassano, G., Tortorella, F. and Zaccaria, G., Classification of food, beverages and perfumes by wo3 thin-film sensors array and pattern recognition techniques, Sensors and Actuators (2001).
[11] Dai, G., A study of the sensing properties of thin film sensor to trimethylamine, Sensors and Actuators B: Chemical, Vol. 53 Nos 1-2, pp. 8-12 (1998).
[12] Pearce, T.C., Schiffman, S.S., Nagle, H.T. and Gardner, J.W. , Handbook of Machine Olfaction, Wiley-VCH, Weinheim (2003).
[13] Dickinson, T.A., White, J., Kauer, J.S. and Walt, D.R., Current trends in artificial-nose technology, Trends in Biotechnology, Vol. 16 No. 6, pp. 250-258 (1998).
[14] Niebling, G. "Identification of gases with classical pattern-recognition methods and artificial neural networks." Sensors and Actuators B: Chemical 18.1 (1994): 259-263.
[15] Wilson, A.D.; Baietto, M. Applications and Advances in Electronic-Nose Technologies. Sensors, 9, 5099-5148 (2009).
[16] R. F. Machado, Detection of lung cancer by sensor array analyses of exhaled breath. Am J Respir Crit Care Med 171: 1286–1291 (2005).
[17] R. Blatt, A. Bonarini, E. Calabro, M. Della Torre, M. Matteucci, U. Pastorino, Lung cancer identification by an electronic nose based on an array of MOS sensors. Neural Networks, IJCNN, pp. 1423–1428 (2007).
[18] Di Natale C., Macagnano A., Martinelli E., Paolesse R., D'Arcangelo G., Roscioni C., Finazzi-Agro A., D'Amico A., Lung cancer identification by the analysis of breath by means of an array of non-selective gas sensors, Biosensors and Bioelectronics, vol. 18, no 10, pp. 1209-1218 (2003).
[19] Chen X., Cao M., Li Y, Hu W., Wang P., Ying K., Pan H., A study of an electronic nose for detection of lung cancer based on a virtual SAW gas sensors array and imaging recognition method, Measurement science & technology, vol. 16, no 8, pp. 1535-1546 (2005).
[20] D. Guo, D. Zhang, N. Li, L. Zhang, J. Yang, A novel breath analysis system based on electronic olfaction. IEEE Trans. Biomed. Eng. 57(11), 2753–2763 (2010).
[21] Arend Kolk et al., Electronic-nose technology in diagnosis of TB patients using sputum samples. J. Clin. Microbiol. (2010).
[22] World Health Organization, Fact sheet 104 (2014).
[23] Brezmes J., Canyellas N., Llobet E., Vilanova X., Application of artificial neural networks to the design and implementation of electronic olfactory system, 5th seminar on Neural Network Applications in Electrical Engineering, NEUREL (2000).
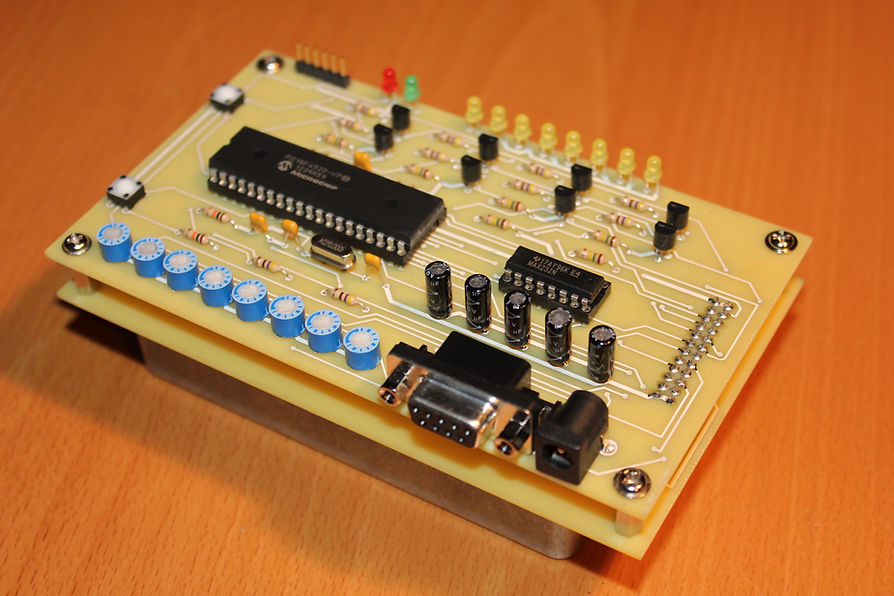